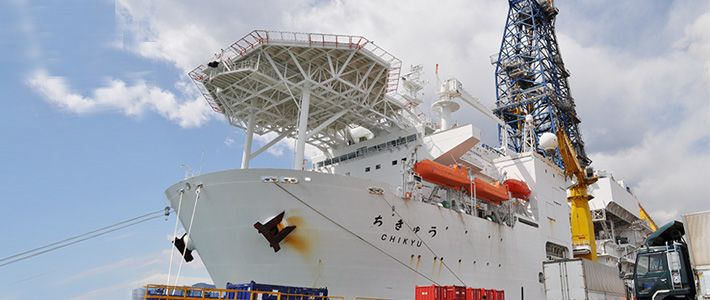
Current Progress in Methane Hydrate Development
Economy Science Technology- English
- 日本語
- 简体字
- 繁體字
- Français
- Español
- العربية
- Русский
An Attractive Energy Resource
The majority of Japan’s natural gas supply comes from liquefied natural gas imported from other countries. In 2011 the amount of annual LNG imports topped 100 billion cubic meters for the first time. The total cost of these imports also reached a record high of ¥4.8 trillion, up 37% from the previous year, due in part to the string of nuclear power plant shutdowns following the Great East Japan Earthquake. The stable supply of natural gas is predicted to increase in the future, with the greater development of shale gas in the United States, but price remains a concern. According to BP's Statistical Review of World Energy (2011), the import price in Japan of LNG (CIF price(*1)) is 2.5 times higher than the Henry Hub price(*2) in the United States; this has a large impact on the unit price of electricity and on Japan’s trade balance.
Given these circumstances, the methane hydrate resources that exist in Japan’s exclusive economic zone are priced attractively for the resource-poor country. Methane gas produced from methane hydrate resources is the major component of natural gas. Thus, if an economical production technology is developed, Japan would be able to not only secure its long-term, stable energy supply but also increase its energy self-sufficiency rate. It would also be a powerful card to play when negotiating LNG imports. Japan’s Ministry of Economy, Trade, and Industry has been promoting the development of methane hydrate resources, and progress has now reached the point where full-fledged efforts leading to marine production tests are set to begin next year.
Burning synthetic core. (Photo: Methane Hydrate Research Center)
Methane hydrate is a clathrate hydrate that contains about 170 m3 of methane gas (standard state conversion) per 1 m3. It is sometimes referred to as “fiery ice” because of its outward resemblance to ice. In nature, methane hydrate can be found under conditions of relatively low temperatures and high pressure. While deposits generally occur in either permafrost zones or ocean regions at the margins of continents, they have also been observed in deep bodies of water such as the Caspian Sea and Lake Baikal. Reports on the total volume of this methane gas have varied considerably, from several hundred trillion cubic meters to ten thousand times that amount. Many recent reports, however, estimate the amount to be several quadrillion cubic meters. Near Japan, bottom-simulating reflectors, indicating the existence of methane hydrate, have been located in an ocean area stretching from the coast of Tokachi from Erimo to Hidaka in Hokkaidō; in the ocean southwest of Sado, offshore from Kashimanada; and in the Pacific Ocean off the coast of Shizuoka Prefecture down to the east coast of Shikoku and Kyūshū. The total area is said to be roughly 122,000 km2.
Methane Hydrate Resource Development
In July 2001 the Ministry of International Trade and Industry (now METI) announced a plan to develop methane hydrate in Japan. In order to implement this plan, the Research Consortium for Methane Hydrate Resources in Japan (also known as MH21 Research Consortium) was established in March 2002. During Phase 1 (2001–8), the amount of primary methane hydrate resources in the eastern Nankai Trough from the Tōkai coast to the seas off Kumano was assessed and shown to be about 1.14 trillion cubic meters. This amount is equivalent to 11 years’ worth of LNG imports to Japan, given the annual consumption level at the time. Also during Phase 1 of the project, a depressurization technique was developed for highly efficient energy production. In addition, fundamental technologies were developed for future commercialization, including technologies to measure and evaluate the properties and degradation characteristics under in situ conditions in methane hydrate layers, a production simulator for development of methane hydrate resources (MH21-HYDRES), and a stratum deformation simulator (COTHMA). To confirm the effectiveness of the depressurization production technique, a verification test was conducted in a permafrost zone located in Canada’s Mackenzie Delta. The six-day test demonstrated that continuous production was possible using the depressurization method. In 2009 this project shifted to Phase 2, implemented by a research consortium that includes the Japan Oil, Gas, and Metals National Corporation and the National Institute of Advanced Industrial Science and Technology.
The methane hydrate seen in nature may be broadly divided into that exposed in large masses near the surface of the seafloor, that which exists in aggregates along fissures in mud layers, and that which exists within pores in sandy layers. The methane hydrate layer that is currently targeted for development is in sandy layers 200 to 300 meters below the sea floor. Methane hydrate layers in such formations are similarly targeted in the United States, because the good permeability of sandy layers allows decomposed methane gas to flow relatively quickly. This makes the layers promising for production of large amounts of gas. Another motivating factor is that much of the conventional oil and gas field development technology that has been accumulated over the years can be applied. Moreover, since methane hydrate exists in pores in sandy layers, few major changes are caused in the structure of the sandy layer itself, even when the methane hydrate is broken down into methane gas and water and then removed. As a result, the possibility of large-scale subsidence and disruption of geological layers is low.
In conventional oil and gas fields, high-pressure gas exists in places such as consolidated sandstone, from which it flows naturally when the sandstone is drilled. Methane hydrate, in contrast, does not flow naturally because it exists as a solid in pore spaces in unconsolidated sandy layers. Therefore, methane hydrate must be broken down and recovered as gas. Other characteristics that set methane hydrate apart from conventional oil and gas fields include the fact that development targets shallow layers in the deep sea, production is accompanied by large changes in the physical properties of the methane hydrate layer, and the temperature of the methane hydrate layer decreases with production.
U
sing the depressurization method, the pressure of the methane hydrate layer is lowered as the water is removed from production wells, the methane hydrate is broken down, and methane is recovered. Decomposition progresses as methane hydrate is heated at a level equivalent to the decomposition heat from the methane hydrate itself and from the surrounding pore water and sand. Production is accompanied by a continual decline in the temperature of the stratum on the heated side. Decomposition is suspended when the production and dissociation equilibrium temperature of methane hydrate is reached in correspondence with the pressure of the depressurized stratum. Because of this, the initial temperature of the stratum is an important factor that determines the production and recovery rates under the depressurization method. The energy needed for production is basically only that necessary to power the pump that draws up underground water. Given this high energy efficiency, depressurization shows promise as a core commercial production technique.
The Path to Commercial Viability
In February 2012 advance drilling for the world’s first production test was conducted at the second Atsumi sea knoll about 70 km south-southeast of the Atsumi Peninsula and roughly 50 km southeast of the Shima Peninsula. One production well and several monitoring wells were drilled. In the production well, the methane hydrate layer will be drilled prior to the production tests in January to March 2013; the well will be completed; and well equipment consisting of a gas separator, electromagnetic water pump, and sensors will be installed. The monitoring wells will be set at intervals of about 20 meters with the aim of measuring how temperature changes in the methane hydrate layer during the production process; and inclinometers and other devices will be installed on the seafloor surface. The measurements obtained from this monitoring can be expected to lead to improvements in the accuracy of the production simulator and the stratum deformation simulator, which were developed previously. One key aspect of the production, which will employ the depressurization production method, is to demonstrate that stable production is possible using this method even for methane hydrate layers located in the ocean.
Since the Great East Japan Earthquake, attention has shifted to methane hydrate as a potential source of natural gas. However, the development of this kind of new resource requires a solid technical foundation obtained through repeated verification tests. Marine production tests are scheduled to be carried out next year; the more of these tests that are conducted, the greater the accuracy of the developed technology. Meanwhile, the basic technology cultivated through the development of methane hydrate resources may have a ripple effect, leading to applications that benefit the development of conventional oil and gas fields. It may also lead to the cultivation of original Japanese technology for the development of resources. In any case, it will be necessary to train the researchers and engineers who will carry this development forward in the future.
(Originally written in Japanese on April 2, 2012.)